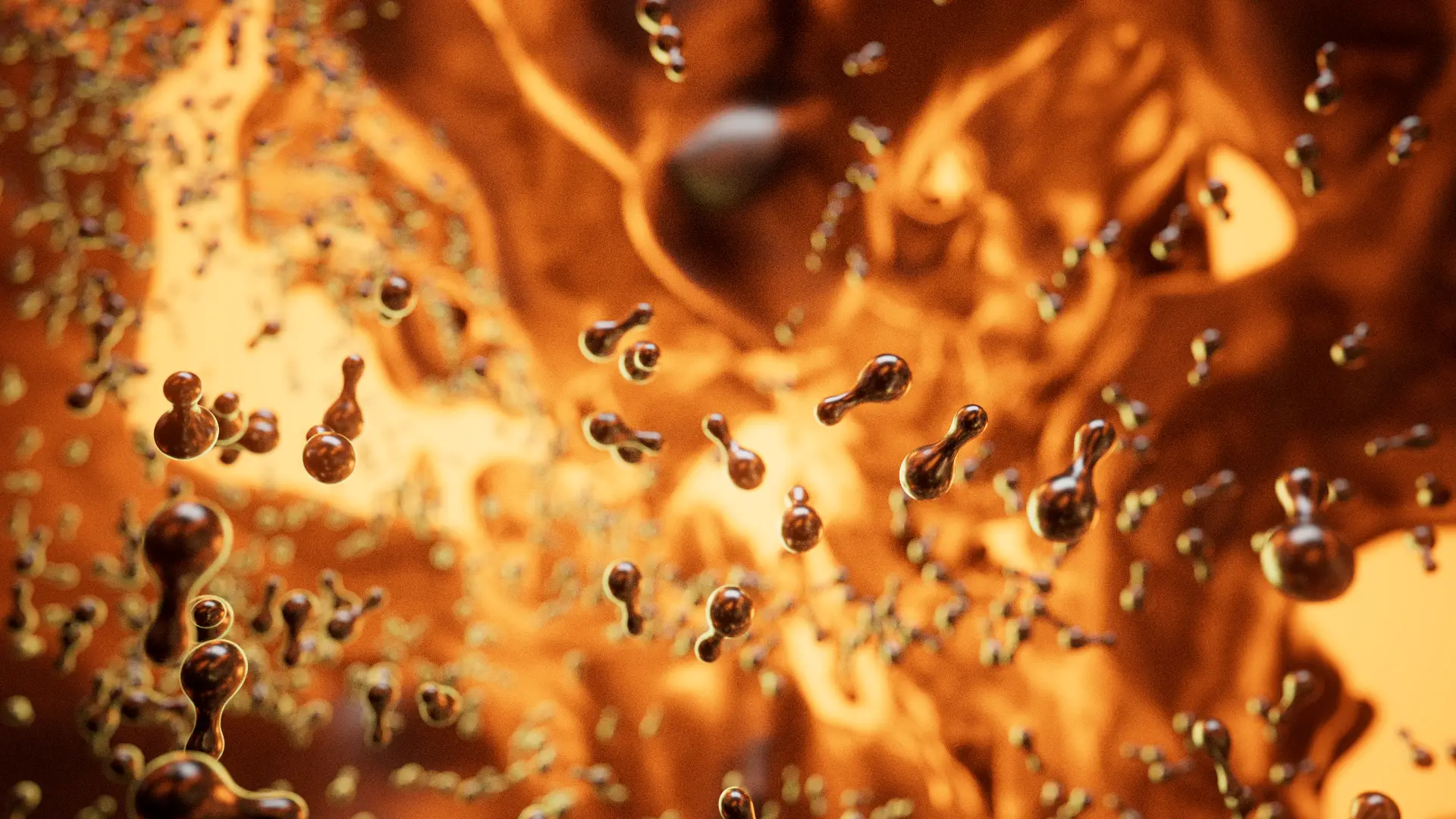
The fundamentals
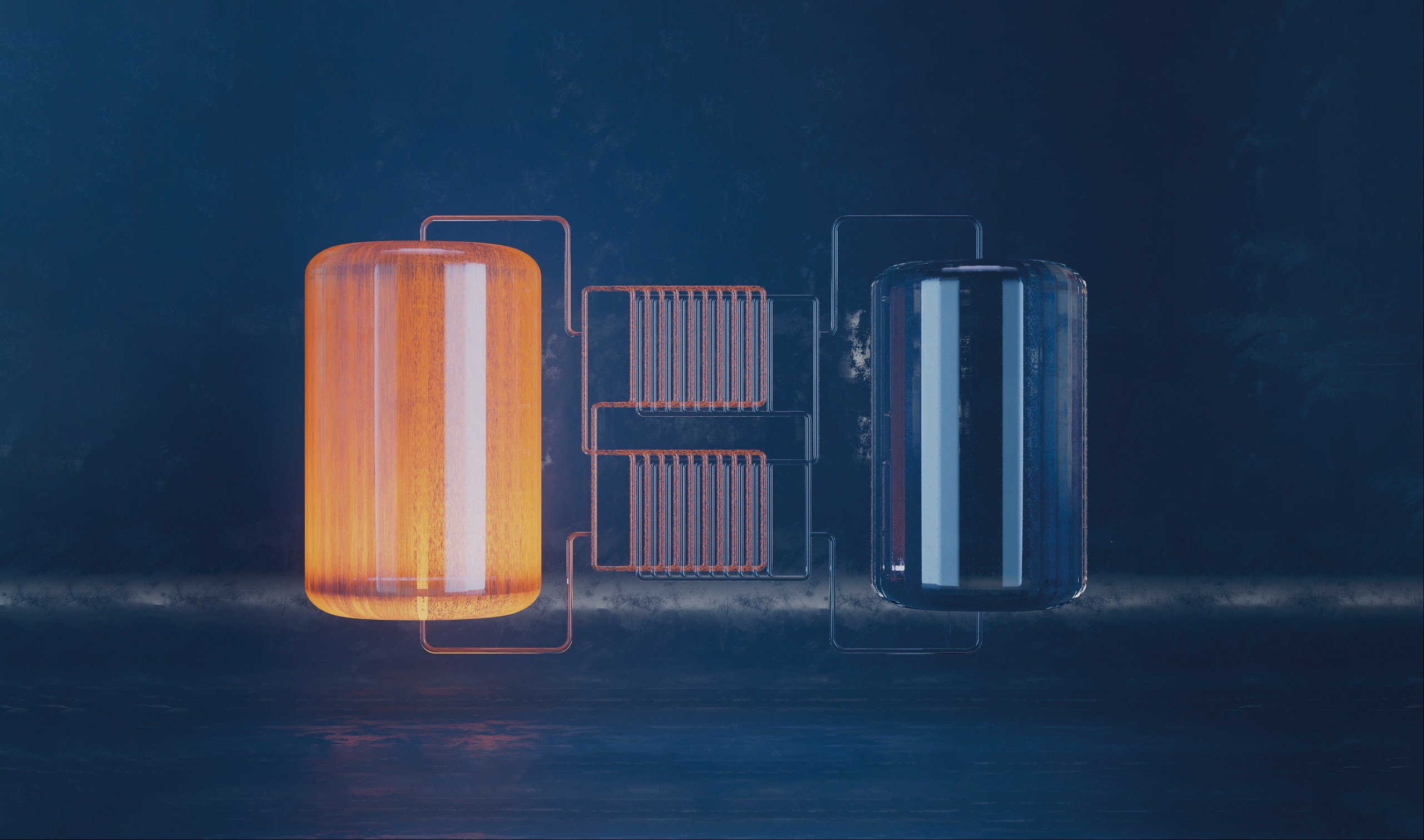
Working principle
Flow batteries were initially developed in the 1960s by The USA’s National Aeronautics and Space Administration, better known simply as NASA. But it wasn’t until the 1980s that their popularity picked up speed, after they were proven to last for more than 10,000 charge/discharge cycles. Along with the continuously growing installed base of renewable energy systems, most notably solar and wind power, it has become obvious that the need to store large, indeed very large, quantities of electrical energy for longer periods of time is growing equally quickly. Such energy storage is essential if we are to achieve a total transition from fossil fuels to renewable energy.
The term flow battery covers a family of storage systems where each one will apply the same fundamental working principle, while using different combinations of active materials. The heart of a flow battery is a so-called electrochemical cell, which is a multi-layer assembly of an ion-selective membrane, catalyst layers and electrodes.
A complete flow battery system, also referred to as a redox flow battery or RFB, is constructed around such electrochemical cells, where chemical energy is provided by the chemical reaction of two active materials. The active materials are contained within the system, separated by the membrane, and circulate in a closed loop, each one in their own respective space.
When an electrical power source is connected, which is when the battery is charging, a chemical redox reaction starts. Ion exchange then occurs through the membrane, resulting in electric current. During discharge, when applying an electrical load, the reverse chemical reaction takes place.
The voltage of the electrochemical cell is determined by the Nernst equation and ranges in practical applications from 1.0 to 2.2 V, depending on the selected active materials.
In order to increase the total electrical power, individual electrochemical cells are stacked, which is another way to say that the cells are electrically interconnected in series. To design systems with (very) large power levels, multiple stack assemblies can be interconnected.
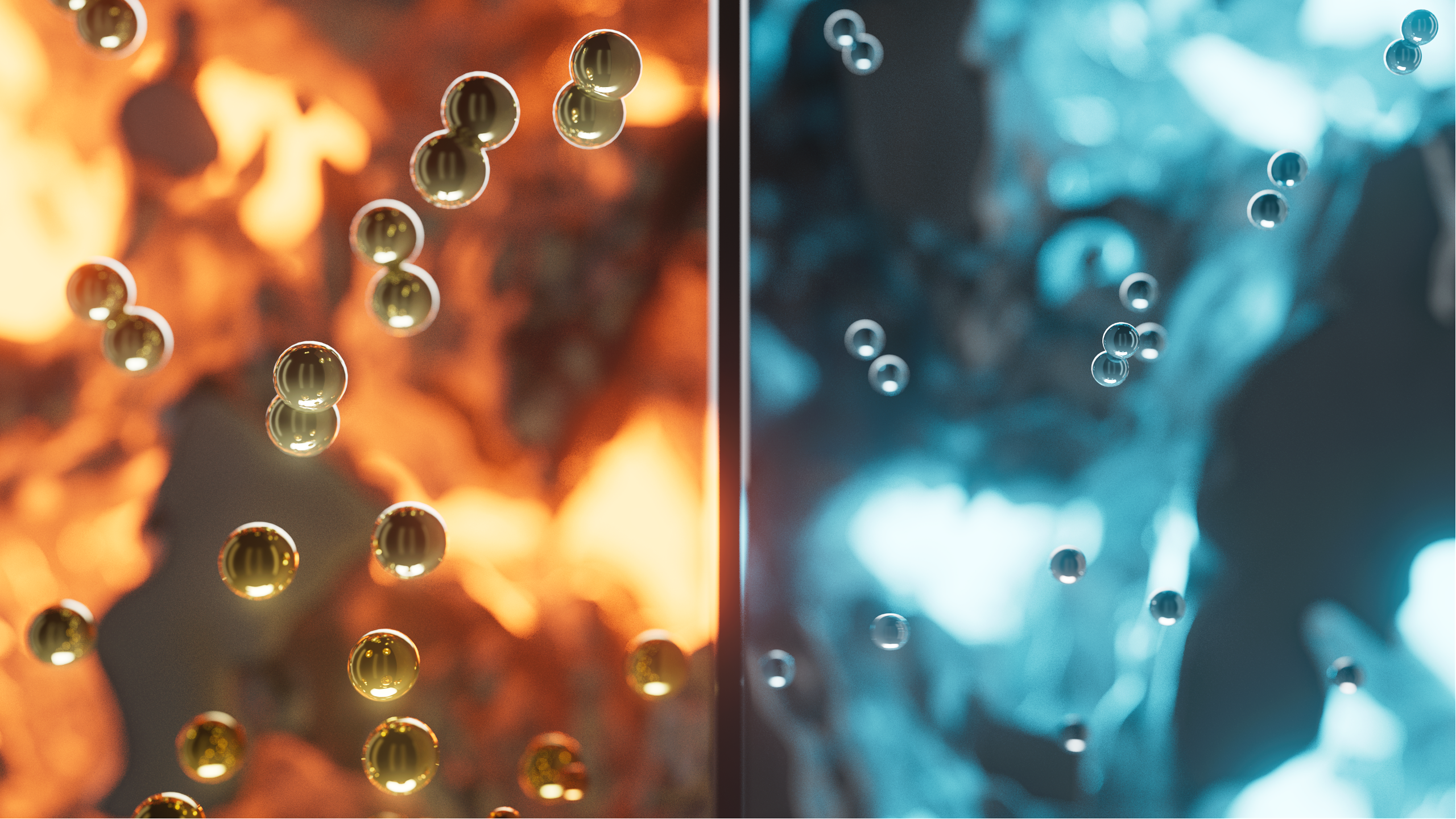
The power [MW] of a flow battery system, as depicted above, is determined by the surface area of the ion-selective membrane, while the capacity [MWh] of the system is determined by the volume of the catholyte and anolyte reservoirs.
The fact that the membrane surface area and the reservoir volumes can be dimensioned individually highlights one of the most distinguishing properties of flow batteries, as opposed to traditional electricity storage systems where power [MW] and capacity [MWh] scale simultaneously.
Long-term performance of hydrogen-bromine flow batteries using single-layered and multi-layered wire-electrospun SPEEK/PFSA/PVDF membranes
Sanaz Abbasiab, Yohanes Antonius Hugob, Zandrie Bornemanac, Wiebrand Koutb and Kitty Nijmeijer*ac
aMembrane Materials and Processes, Department of Chemical Engineering and Chemistry, Eindhoven University of Technology, P.O. Box 513, 5600 MB Eindhoven, The Netherlands. E-mail: D.C.Nijmijer@tue.nl
bElestor BV P.O. Box 882, 6800 AW Arnhem, The Netherlands
cDutch Institute for Fundamental Energy Research (DIFFER), P.O. Box 6336, 5600 HH Eindhoven, The Netherlands
Abstract
Sulfonated poly (ether ketone) (SPEEK), perfluorosulfonic acid (PFSA), and polyvinylidene fluoride (PVDF) were wire-electrospun. Subsequently, multiple electrospun layers in different arrangements were hot-pressed into sustainable membranes for use in hydrogen-bromine flow batteries (HBFBs). The relationship between the electrospun layer composition and arrangement, membrane properties, and battery performance was explored. Wire-electrospinning and hot-pressing improved SPEEK and PFSA/PVDF compatibility, yielding dense membranes. Higher SPEEK contents lead to rougher morphologies, while the insulating nature of PVDF decreases the ion exchange capacity (IEC) and HBr uptake compared to commercial PFSA. The multi-layer assembly negatively impacted the membrane transport properties compared to the single-layer arrangement. Although wire-electrospinning improves the polymer dispersion and fixed charge density, SPEEK-rich regions of the blend membranes lack the high selectivity of PFSA, thus reducing the ionic conductivity. This is especially clear in the multi-layer membranes with accumulated SPEEK in the intermediate layer in the through-plane direction. Following initial property comparisons, thinner wire-electrospun SPEEK membranes were prepared with area resistance in the PFSA-comparable range. Among the wire-electrospun SPEEK/PFSA/PVDF membranes, the single-layered membrane with 8 wt% SPEEK (SPF1-8; 62 μm) displayed stable HBFB performance at 200 mA cm−2 over 100 cycles (64 cm2 active area). Based on the ex-situ measurements and cell performance results, a total of ∼10.5 wt% SPEEK is suggested as the limit for both single and multi-layered wire-electrospun membranes, combined with a maximum membrane thickness of ∼50 μm. This ensures robust HBFB performance, positioning wire-electrospun SPEEK/PFSA/PVDF membranes as a PFSA alternative in energy storage.
Wire based electrospun composite short side chain perfluorosulfonic acid/ polyvinylidene fluoride membranes for hydrogen-bromine flow batteries
Yohanes Antonius Hugo a, b, Wiebrand Kout b, Antoni Forner-Cuenca a, Zandrie Borneman a, c, Kitty Nijmeijer a, c, *
a Membrane Materials and Processes, Department of Chemical Engineering and Chemistry, Eindhoven University of Technology, PO Box 513, 5600MB Eindhoven, the Netherlands
b Elestor B.V., 6827 AV Arnhem, the Netherlands
c Dutch Institute for Fundamental Energy Research (DIFFER), P.O. Box 6336, 5600 HH Eindhoven, the Netherlands
⁎ Corresponding author. E-mail address: d.c.nijmeijer@tue.nl (K. Nijmeijer).
Abstract
A main component of a hydrogen-bromine flow battery (HBFB) is the ion exchange membrane. Available membranes have a trade-off between the major requirements: high proton conductivity, low bromine species crossover, and high mechanical and chemical stability. To overcome this, electrospinning of a highly proton conductive polymer (short side chain perfluorosulfonic acid (SSC PFSA)) and a hydrophobic inert polymer (polyvinylidene fluoride (PVDF)) was used to electrospin composite polymer fiber mats. Piles of multiple mats were hot pressed resulting in dense ion exchange membranes. Membranes with three different SSC PFSA/PVDF ratios were prepared, characterized, and subjected to short and long term (1500 h) HBFB testing. The electrospun membranes have performances very comparable to those of commercial membranes. For the SSC PFSA/PVDF electrospun membrane, a higher SSC PFSA loading gives a higher membrane proton conductivity compared to a lower loading, but at the expense of a higher bromine species crossover. The SSC PFSA/PVDF (50/50 wt%) membrane shows a coulombic efficiency of 98%, a voltaic efficiency of 80% and an initial available capacity of 105 Ah L− 1 at a current density of 150 mA cm− 2, which equals that of the current benchmark long side chain PFSA membrane. This performance is constant over 200 cycles during 2 months of continuous HBFB operation.
High selectivity-conductivity reinforced perfluorosulfonic acid membranes for hydrogen-bromine flow batteries.
Yohanes Hugo1, 2, Wiebrand Kout1, Friso Sikkema1, Zandrie Borneman2, Kitty Nijmeijer2
1Elestor B.V., 6812 AR Arnhem, the Netherlands
2Membrane Materials and Processes, Eindhoven University of Technology, Department of Chemical Engineering and Chemistry, PO box 513, 5600 MB Eindhoven, The Netherlands
Abstract
Reinforced proton exchange membrane (PEM) were developed to increase the mechanical strength of thin membranes (≤30 µm) for PEM fuel cell applications. In hydrogen-bromine flow batteries (HBFBs), Br2 and Br– crossover through the membrane may affect the lifetime of HBFBs as a result of dissolution or passivation of the platinum catalyst. One study suggested that the reinforcement reduces the bulk Br2 and Br– transport and x-y (in-plane) swelling [1].
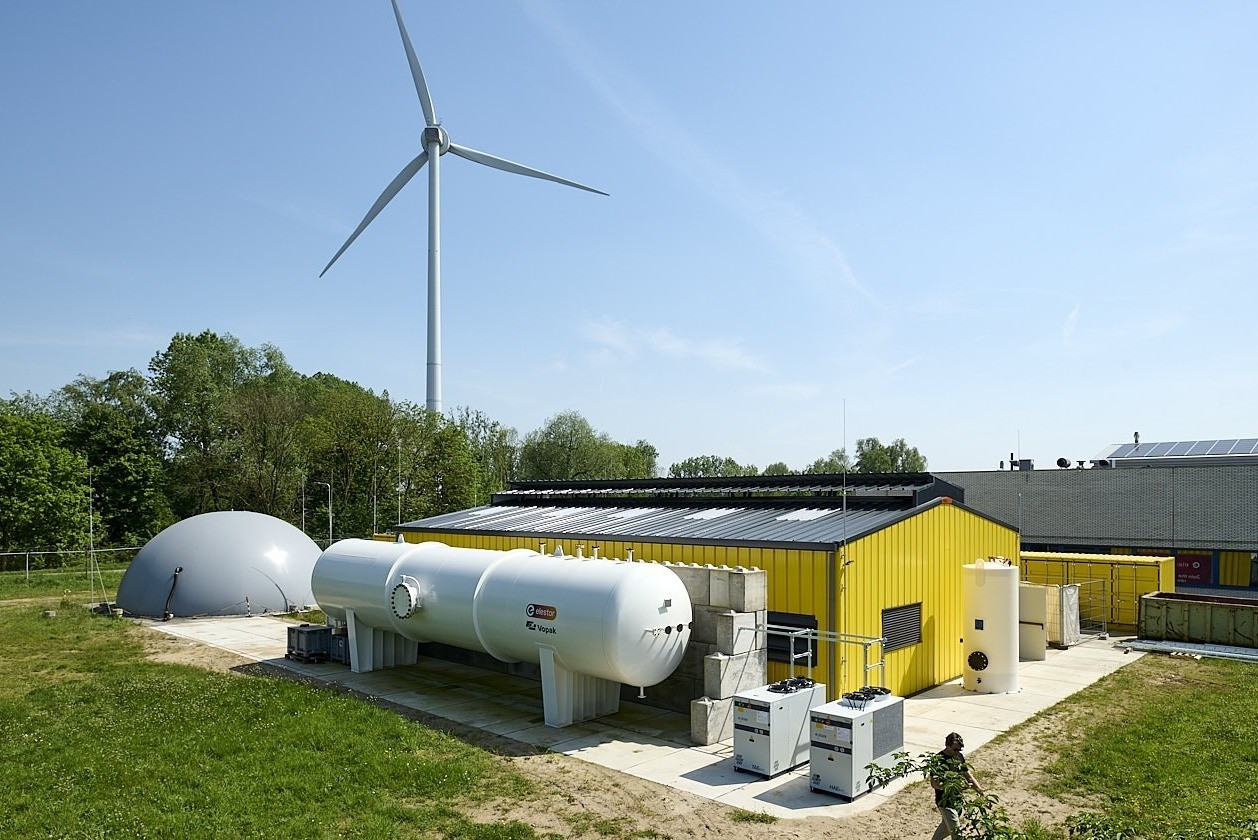
One year since winning Offshore Wind Innovators Awards
Elestor covered by TKI Offshore Energy: "How's Elestor faring, one year after winning both the Offshore Wind Innovators Jury and Audience Awards? We have a look at the innovators of renewable energy storage and the progress they made. Elestor offers an important element for a successful energy transition."
Read more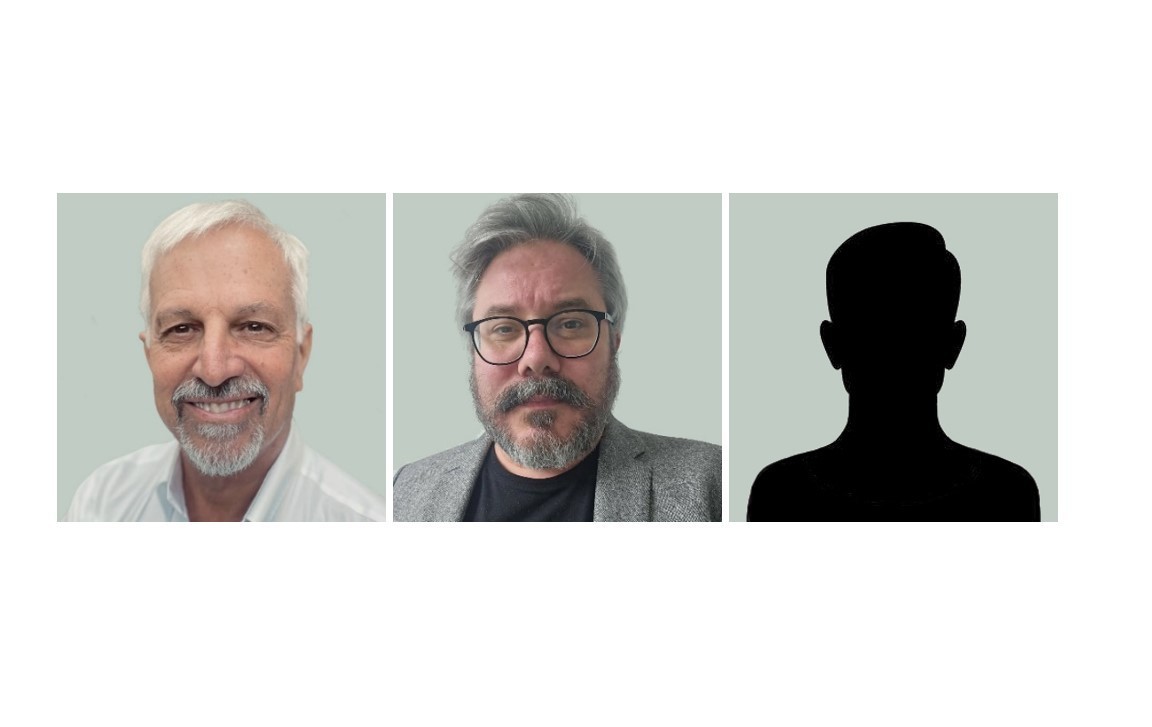
Elestor secures top international scientists for its Technical Advisory Board
Arnhem, The Netherlands, May 21, 2024. Dutch long-duration electricity storage company Elestor has secured the participation of a prominent group of scientists and sector experts as members of its newly created Technical Advisory Board. “As a cutting edge technology company, we invest significant resources in research and development in order to ensure we remain ahead of rivals in the clean energy storage sphere,” said Guido Dalessi, CEO, Elestor. “This leadership position has enabled us to attract top international scientists to our Technical Advisory Board, which is obviously going to be 100% independent to preserve the integrity of its members and of their conclusions.”
Read more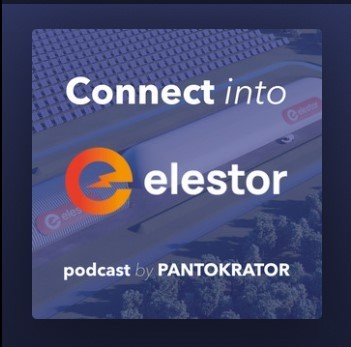
LDES: Hydrogen Flow Batteries with Guido Dalessi, CEO Elestor
For this podcast episode, we have a special guest, Guido Dalessi, CEO of Elestor. Listen as we delve into their unique Hydrogen-Bromine flow batteries, discuss LDES in the Europe, how to secure right partnerships, and Elestor's plans for growth. Kudos to {antokrator ! https://www.pantokratorltd.com/
Read more