.png?)
Cost of storage
Defining cost of storage
To determine whether Elestor’s mission - Reducing electricity storage costs to the absolute minimum - is indeed accomplished, it is important to have a common understanding of the definition of Cost of Storage. This obviously goes beyond simply considering the investment costs (Capex) for a particular storage system.
Cost of Storage is a very important concept because, in essence, the figure determines the economic value of a storage technology, and thus of its market adoption, and finally of its impact on the energy transition.
Over the years, Cost of Storage has been quantified in several ways. Today, and particularly with flow batteries coming to the fore, the terminology as well as the mathematical approach needs some adjustment.
Levelised Cost of Storage (LCoS)
To objectively compare different storage technologies from an economic point of view, the so-called Levelised Costs of Storage, or LCoS, has been introduced. The LCoS says potentially what the bottom line costs are for storing 1 MWh, thereby taking several system characteristics into account.
In other publications, the LCoS is presented in various forms, whereby the differences are mainly found in the number of parameters that are considered in the calculation. Another scientific approach, taking many detailed parameters into account, is given by Lazard.
In its most simplified and traditional form, the LCoS calculation only considers the Investment cost per MWh’ [€/MWh], the lifetime of the storage system [cycles], and its typical Roundtrip (dis)charge efficiency [%], as shown below. This approach has been a proper and workable way to objectively compare the storage cost per MWh of different technologies. And this was indeed true for a long time – until flow battery technology started gaining popularity.
While the investment cost per MWh is a fixed figure for traditional technologies, this is different for flow batteries. After all, flow batteries can be designed with virtually any combination of power [MW] and capacity [MWh].
The investment cost for such systems is not only a function of their capacity [MWh], but also of their power [MW]. Therefore, above LCoS calculation cannot be applied in this form for flow batteries.
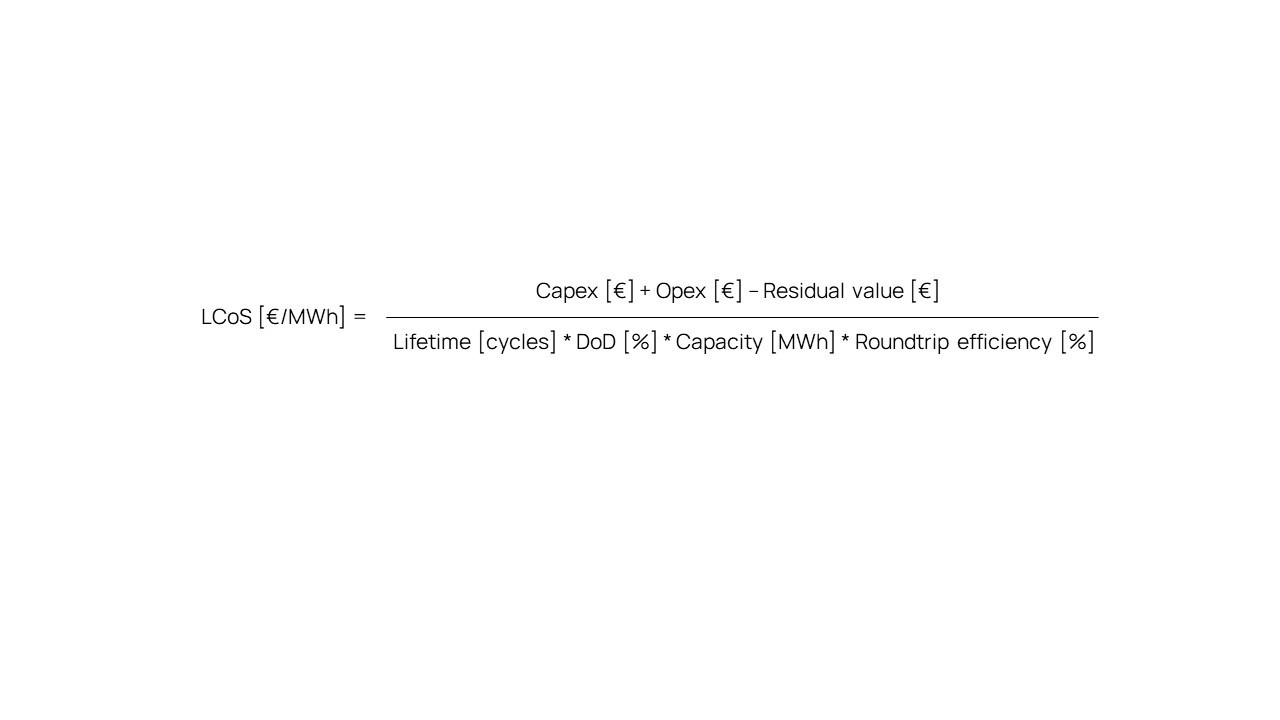
For flow batteries, the investment costs per MWh is not a fixed number
If, for instance, doubling the storage capacity of a traditional battery is desired, then the power is also doubled, automatically. In fact, a second complete storage unit is added in this case, obviously also resulting in double the investment cost (Capex).
Since power [MW] and capacity [MWh] of traditional batteries have a fixed ratio, the investment cost can simply be expressed in a fixed number of € per MWh.
The Capex of such systems is then calculated by multiplying this constant by the desired storage capacity.
This is different for flow batteries. With flow batteries, doubling the storage capacity [MWh] simply means a doubling of the volumes of active materials. The system’s power remains unchanged and this then obviously does not result in double investment cost.
The additional costs for doubling the capacity of Elestor’s solution are only marginal, because the active materials (H2 and Br2) were deliberately selected for their low cost.
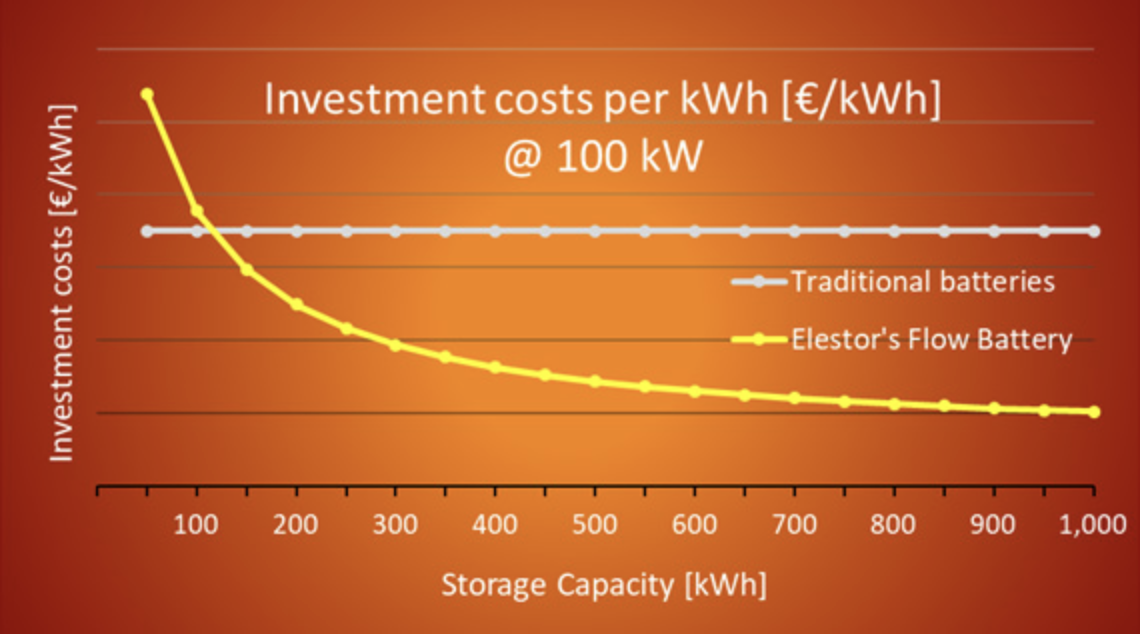
Confused? Here is a different approach.
A practical, straight forward approach is to calculate the ratio of the total investment cost for a system and the total amount of electric energy [MWh] that this system delivers during its lifetime:
This calculation fundamentally leads to a true cost figure per MWh, whereby the most dominant factors are considered. Furthermore, it allows an objective comparison of all kinds of storage technologies, traditional and contemporary.
The approach presented on this page is deliberately simplified in order to explain the dominant factors determining the LCoS. Elestor would be delighted to provide an accurate LCoS calculation for specific storage applications, so please get in touch if this is what you’re looking for.
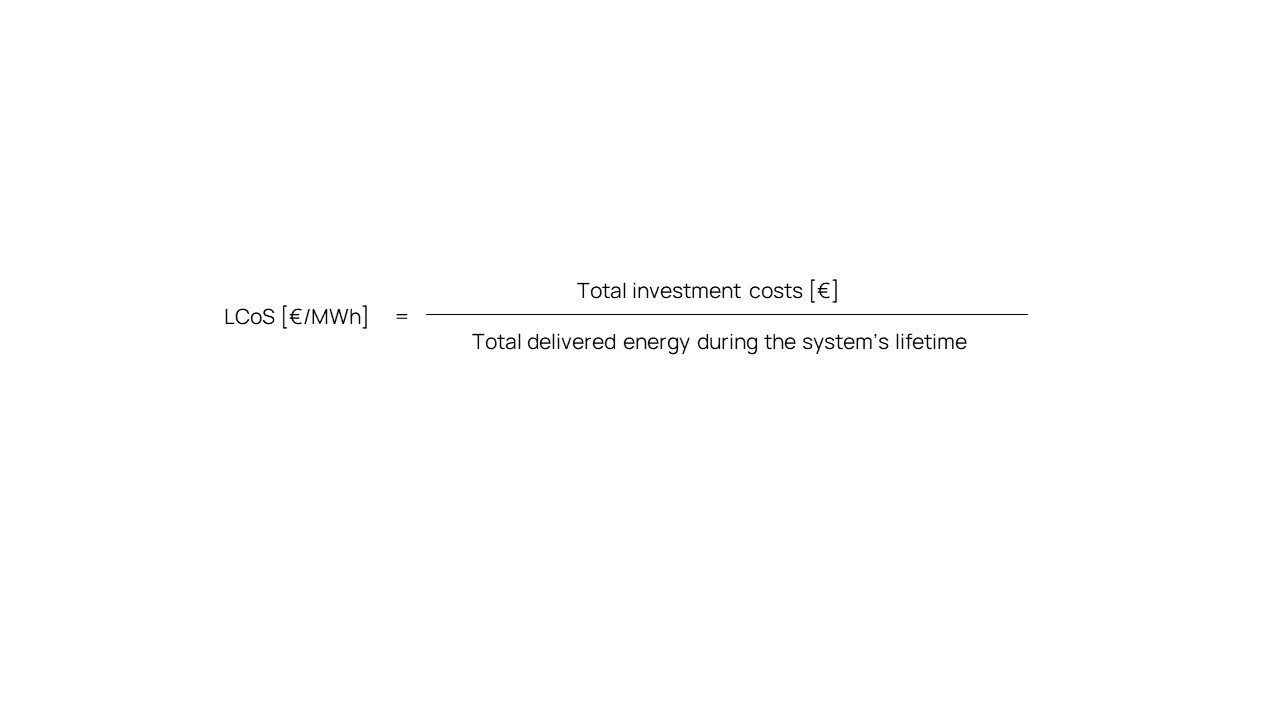
Stack replacement gives flow batteries a full second life
Going into more detail, operational expenses (Opex) and residual value can also be considered. The numerator of above formula then becomes the sum of Capex and Opex, minus the residual value of the system at the end of its life. Especially for Elestor’s battery, this residual value is a number with great relevance: During its lifetime, the electrochemical storage process does not degrade and therefore none of the chemicals are consumed. As a result, a large part of the storage system keeps its original value. Because of the fact that the HBr battery is truly accessible for service, upgrades and refurbishment, cell stacks can easily be replaced at the end of their lives. This gives the flow battery a full second life.
The residual value of a hydrogen bromine flow battery system is therefore significant. Including the residual value in the cost calculation for Elestor’s flow batteries results in considerably lower figures for LCoS than the costs displayed in the graph.
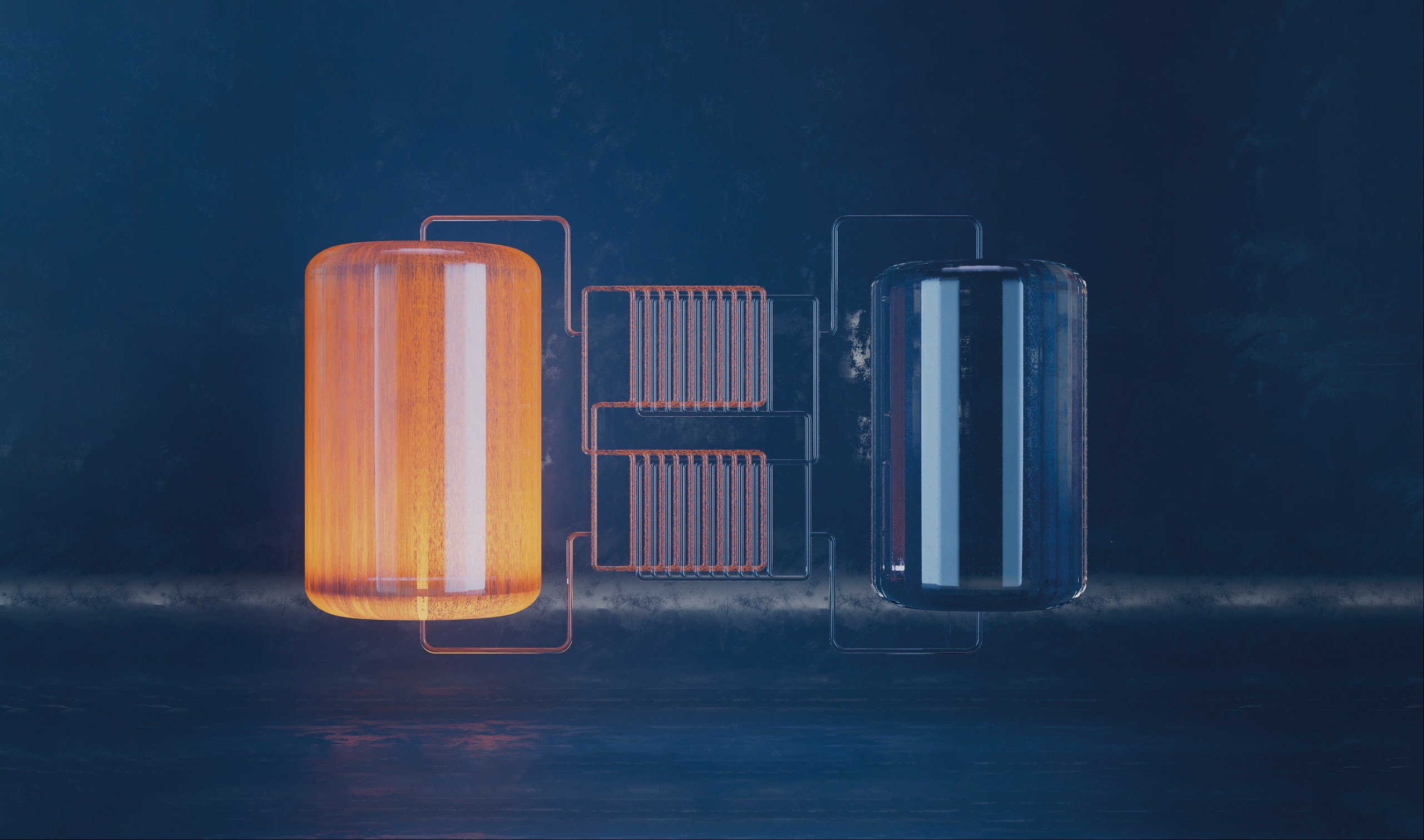
A flow battery’s lifetime does not depend on depth of discharge
Last but not least, the figure for ‘Capacity [MWh]’ must be interpreted as the practically usable capacity, which is not necessarily the same as the purchased capacity.
Traditional storage technologies do generally not allow full charge/discharge between 0% and 100% without compromising the system’s lifetime. In other words, if a traditional battery was to be used between 0% and 100%, then its lifetime would be dramatically shortened.
In battery specifications, this phenomenon is referred to as depth of discharge or DoD [%], which describes the maximum allowed charge and discharge level. The following graph shows the relation between DoD and the lifetime of traditional storage systems.
For traditional batteries, the practical DoD is roughly in the range of 70%, as these batteries are commonly operated between around 15% and 85% state-of-charge. This is done in order to prevent a significant lifetime reduction, which would occur with a larger DoD.
Flow batteries, however, allow a DoD of virtually 100% without it affecting its lifetimes. DoD has a great impact on LCoS calculations, and is therefore a very relevant figure:
The in-practice usable storage capacity is equal to DoD* purchased capacity.
As such, a DoD of 70% immediately results in a 50% higher LCoS, because only 70% of the purchased capacity is practically used. Including the DoD effect further enlarges the difference in LCoS between traditional storage systems and flow batteries.
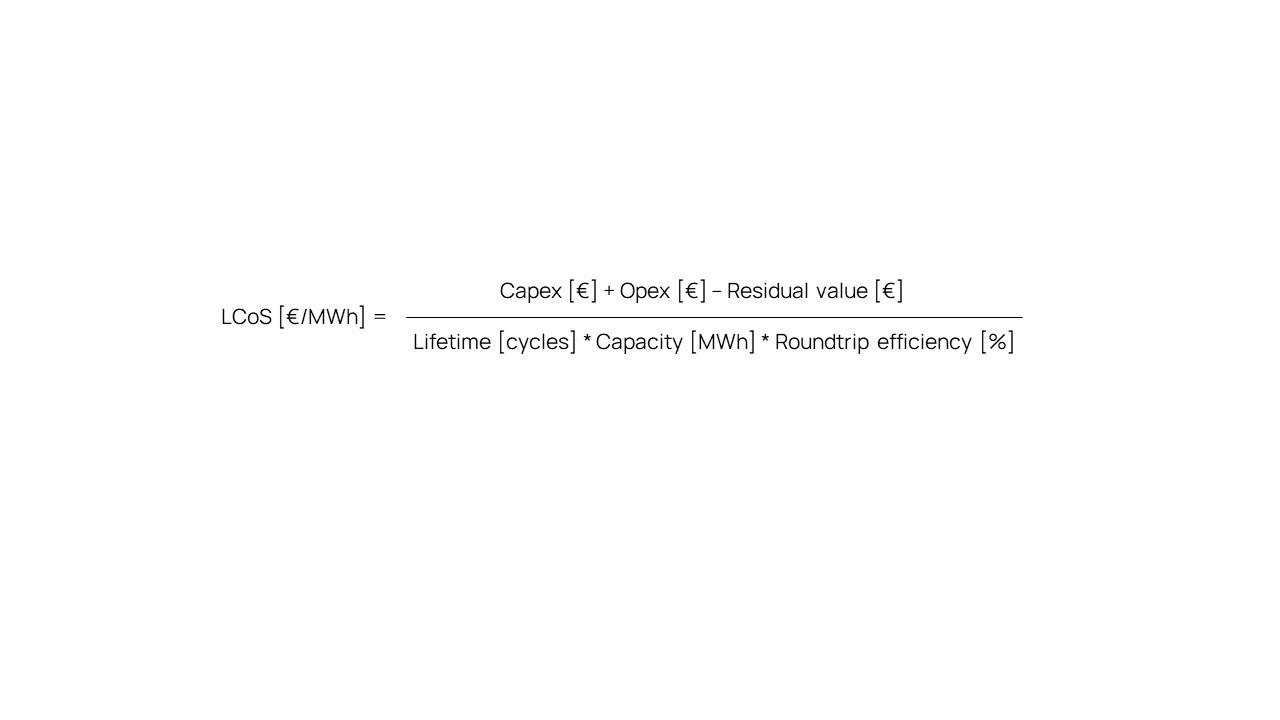
Long-term performance of hydrogen-bromine flow batteries using single-layered and multi-layered wire-electrospun SPEEK/PFSA/PVDF membranes
Sanaz Abbasiab, Yohanes Antonius Hugob, Zandrie Bornemanac, Wiebrand Koutb and Kitty Nijmeijer*ac
aMembrane Materials and Processes, Department of Chemical Engineering and Chemistry, Eindhoven University of Technology, P.O. Box 513, 5600 MB Eindhoven, The Netherlands. E-mail: D.C.Nijmijer@tue.nl
bElestor BV P.O. Box 882, 6800 AW Arnhem, The Netherlands
cDutch Institute for Fundamental Energy Research (DIFFER), P.O. Box 6336, 5600 HH Eindhoven, The Netherlands
Abstract
Sulfonated poly (ether ketone) (SPEEK), perfluorosulfonic acid (PFSA), and polyvinylidene fluoride (PVDF) were wire-electrospun. Subsequently, multiple electrospun layers in different arrangements were hot-pressed into sustainable membranes for use in hydrogen-bromine flow batteries (HBFBs). The relationship between the electrospun layer composition and arrangement, membrane properties, and battery performance was explored. Wire-electrospinning and hot-pressing improved SPEEK and PFSA/PVDF compatibility, yielding dense membranes. Higher SPEEK contents lead to rougher morphologies, while the insulating nature of PVDF decreases the ion exchange capacity (IEC) and HBr uptake compared to commercial PFSA. The multi-layer assembly negatively impacted the membrane transport properties compared to the single-layer arrangement. Although wire-electrospinning improves the polymer dispersion and fixed charge density, SPEEK-rich regions of the blend membranes lack the high selectivity of PFSA, thus reducing the ionic conductivity. This is especially clear in the multi-layer membranes with accumulated SPEEK in the intermediate layer in the through-plane direction. Following initial property comparisons, thinner wire-electrospun SPEEK membranes were prepared with area resistance in the PFSA-comparable range. Among the wire-electrospun SPEEK/PFSA/PVDF membranes, the single-layered membrane with 8 wt% SPEEK (SPF1-8; 62 μm) displayed stable HBFB performance at 200 mA cm−2 over 100 cycles (64 cm2 active area). Based on the ex-situ measurements and cell performance results, a total of ∼10.5 wt% SPEEK is suggested as the limit for both single and multi-layered wire-electrospun membranes, combined with a maximum membrane thickness of ∼50 μm. This ensures robust HBFB performance, positioning wire-electrospun SPEEK/PFSA/PVDF membranes as a PFSA alternative in energy storage.
Wire based electrospun composite short side chain perfluorosulfonic acid/ polyvinylidene fluoride membranes for hydrogen-bromine flow batteries
Yohanes Antonius Hugo a, b, Wiebrand Kout b, Antoni Forner-Cuenca a, Zandrie Borneman a, c, Kitty Nijmeijer a, c, *
a Membrane Materials and Processes, Department of Chemical Engineering and Chemistry, Eindhoven University of Technology, PO Box 513, 5600MB Eindhoven, the Netherlands
b Elestor B.V., 6827 AV Arnhem, the Netherlands
c Dutch Institute for Fundamental Energy Research (DIFFER), P.O. Box 6336, 5600 HH Eindhoven, the Netherlands
⁎ Corresponding author. E-mail address: d.c.nijmeijer@tue.nl (K. Nijmeijer).
Abstract
A main component of a hydrogen-bromine flow battery (HBFB) is the ion exchange membrane. Available membranes have a trade-off between the major requirements: high proton conductivity, low bromine species crossover, and high mechanical and chemical stability. To overcome this, electrospinning of a highly proton conductive polymer (short side chain perfluorosulfonic acid (SSC PFSA)) and a hydrophobic inert polymer (polyvinylidene fluoride (PVDF)) was used to electrospin composite polymer fiber mats. Piles of multiple mats were hot pressed resulting in dense ion exchange membranes. Membranes with three different SSC PFSA/PVDF ratios were prepared, characterized, and subjected to short and long term (1500 h) HBFB testing. The electrospun membranes have performances very comparable to those of commercial membranes. For the SSC PFSA/PVDF electrospun membrane, a higher SSC PFSA loading gives a higher membrane proton conductivity compared to a lower loading, but at the expense of a higher bromine species crossover. The SSC PFSA/PVDF (50/50 wt%) membrane shows a coulombic efficiency of 98%, a voltaic efficiency of 80% and an initial available capacity of 105 Ah L− 1 at a current density of 150 mA cm− 2, which equals that of the current benchmark long side chain PFSA membrane. This performance is constant over 200 cycles during 2 months of continuous HBFB operation.
High selectivity-conductivity reinforced perfluorosulfonic acid membranes for hydrogen-bromine flow batteries.
Yohanes Hugo1, 2, Wiebrand Kout1, Friso Sikkema1, Zandrie Borneman2, Kitty Nijmeijer2
1Elestor B.V., 6812 AR Arnhem, the Netherlands
2Membrane Materials and Processes, Eindhoven University of Technology, Department of Chemical Engineering and Chemistry, PO box 513, 5600 MB Eindhoven, The Netherlands
Abstract
Reinforced proton exchange membrane (PEM) were developed to increase the mechanical strength of thin membranes (≤30 µm) for PEM fuel cell applications. In hydrogen-bromine flow batteries (HBFBs), Br2 and Br– crossover through the membrane may affect the lifetime of HBFBs as a result of dissolution or passivation of the platinum catalyst. One study suggested that the reinforcement reduces the bulk Br2 and Br– transport and x-y (in-plane) swelling [1].
Performance mapping of cation exchange membranes for hydrogen-bromine flow batteries for energy storage
Yohanes Antonius Hugo a, b, Wiebrand Kout b, Antoni Forner-Cuenca a, Zandrie Borneman a, c, Kitty Nijmeijer a, c, *
a Membrane Materials and Processes, Department of Chemical Engineering and Chemistry, Eindhoven University of Technology, PO Box 513, 5600MB Eindhoven, the Netherlands
b Elestor B.V., 6827 AV Arnhem, the Netherlands
c Dutch Institute for Fundamental Energy Research (DIFFER), P.O. Box 6336, 5600 HH Eindhoven, the Netherlands
⁎ Corresponding author. E-mail address: d.c.nijmeijer@tue.nl (K. Nijmeijer).
Abstract
Electricity storage is essential for the transition to sustainable energy sources. Hydrogen-bromine flow batteries (HBFBs) are promising cost-effective energy storage systems. In HBFBs, proton exchange membranes are required to separate the two reactive materials, enabling proton transport for charge balancing. In this paper, we present a comprehensive overview of the key properties and an experimental performance map of cation exchange membranes for HBFBs. Our study shows that membrane water uptake is an important property due to its strong correlation with membrane resistance and bromide species crossover. Long chain perfluorosulfonic acid (LC PFSA) membranes are shown to have a better power density–crossover tradeoff and a higher stability than other types of functionalized membranes. The good power density-crossover tradeoff of LC PFSA membranes is the result of the high level of separation of hydrophobic and hydrophilic domains in the membrane, leading to well-connected ionic pathways for proton transport. Reinforcement of long chain LC PFSA membranes further improves their tradeoff because it mechanically constrains the swelling (lower water uptake), resulting in a lower crossover but a similar peak power density. Consequently, reinforced LC PFSA membranes are the most promising option for HBFBs.
Effect of Bromine Complexing Agents on Membrane Performance in Hydrogen Bromine Flow Batteries
Yohanes Antonius Hugo1,2, Natalia Mazur2, Wiebrand Kout2, Guido Dalessi2, Antoni Forner-Cuenca1, Zandrie Borneman1, 3 and Kitty Nijmeijer1,3,*
1Membrane Materials and Processes, Department of Chemical Engineering and Chemistry, Eindhoven University of Technology, P.O. Box 513, 5600 MB Eindhoven, The Netherlands; yohanes.hugo@elestor.nl (Y.A.H.); a.forner.cuenca@tue.nl (A.F.-C.); z.borneman@tue.nl (Z.B.)
2 Elestor B.V., P.O. Box 882, 6800 AW Arnhem, The Netherlands; wiebrand.kout@elestor.nl (W.K.); guido.dalessi@elestor.nl (G.D.)
3 Dutch Institute for Fundamental Energy Research (DIFFER), P.O. Box 6336, 5600 HH Eindhoven, The Netherlands
Abstract
The addition of bromine complexing agent (BCA) to bromine electrolyte is an accepted method to reduce bromine vapor pressure making bromine-based flow batteries inherently safer. It is well-known that the amine functional group of the BCAs interact with Nafionmembranes.The novelty of the current work is that it investigates how this interaction of BCA with the four different membrane chemistries impacts the membrane characteristics and performance of hydrogen bromine flowbatteries(HBFBs). The impact of BCA 13 on the system performance is determined by the membrane chemistry. Exposure of Nafion membranes to BCA leads to 60% higher cell resistance, and 55% lower cell power density at 0.5V at 50% state-of-charge(SOC). This decrease is caused by the strong interaction between the negatively charged sulfonic acid groups in the membrane and the positively charged BCA. Lower SOC, lower bromine concentration and a higher free BCA concentration is detrimental in the cell operation. The use of LC PFSA membranes in the presence of BCA ions should be avoided. while BCA in combination with grafted sulfonated polyvinylidene fluoride (SPVDF) or grafted sulfonated polyethylene (SPE) membranes promising HBFB results are obtained.